AZoRobotics speaks with Yoshiyuki Kageyama from Hokkaido University about his research into creating molecular microrobots that simulate the capabilities of living organisms. Kageyama and his team successfully made a microcrystal that uses self-continuous reciprocating motion for self-propulsion.
The fantasy of microrobotics has been addressed in science fiction for decades. What would you say inspired your research into making this fantastical concept into a reality?
I believe that many of our human fantasies, ideals and dreams are derived from the real-life world. In Japan, where I live, there is a thriving anime culture, and all of them are developed from human society. In there, even inorganic things are depicted as if they are alive.
The essence of my research may be similar to this. I am researching how to make inanimate materials behave like living things. Using my skills in chemistry, and organic chemistry, in particular, to create molecular systems that continue to do things on a tangible scale, which is characteristic of life. This is the theme of my research theme.
When I was a postdoctoral researcher, I worked on the creation of autocatalytic self-production of molecular assemblies that mimic the self-replicating cell division of life. This is an artificial system in which molecular assemblies grow and divide continuously as long as nutrients are supplied. After I was appointed to the current position, I was allowed to organize my own projects, and I started to create new molecular systems that show continuous macroscopic motion.
One aspect of fabricating these robots is self-propulsion – the ability to move self-sustainably. Can you give an overview of how this concept works and how you aimed to achieve this?
The aspect of self-propulsion is a crucial point. There are two methods of propulsion for an object: passive propulsion and self-propulsion.
Passive propulsion is a phenomenon in which an object moves when the environment around the object is changed. The propulsion is fully controlled by its environment. Self-propulsion, on the other hand, is a phenomenon in which an object moves by itself. At the same time, it accepts and consumes its convertible energy, which exists in the system even if the environment is in a steady condition.
The direction and speed of the movement are due to the object and its properties. A self-propulsive object can also move passively, whereas an object without self-propulsion capabilities can only move passively.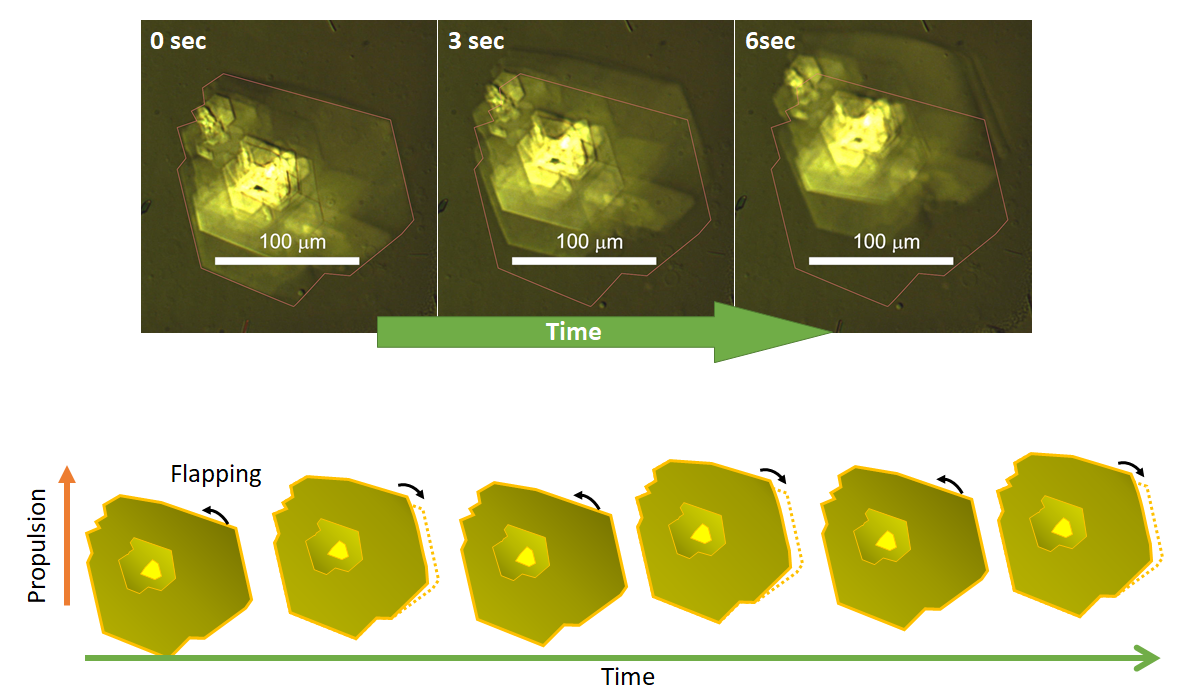
Small self-propulsive objects have been described as solid objects, such as Janus particles, and objects of indeterminate shape, such as oil droplets. They use ingested energy to create a potential gradient around them and slide down the slope.
In contrast, our robot is designed to swim like an animal. In other words, it is a robot that moves through water in a stroke-like manner.
Did you come across any challenges when attempting to achieve self-propelling microrobots, and if so, how did you overcome them?
We came across two general challenges in our attempt to realize a swimming molecular robot. The first is to create a self-sustaining shape change. Continuous motion is a phenomenon that Erwin Schrödinger described as characteristic of life. Subsequent developments in science have shown that it is not unique to life, but chemists still agree that it is not easy to achieve artificially.
I overcame this problem by taking a different approach from the general chemical approach, which solved the challenge by tuning and sophisticating molecular-level structure.
It is well known that both fluorescent and catalytic molecules change their shape and then return to their original structure by releasing heat energy or products, and the process is then repeated. Thus, molecules are inherently self-sustaining.
The interpretation that non-living organisms are not self-sustaining is simply that objects made up of many molecules do not appear to be in continuous motion. My point of view is that to create self-sustained motion, you need to make it visible. To make it visible, the sustaining movement of molecules needs to be compiled into a collective object. By changing my point of view from that of other researchers, I changed my research methodology and consequently overcame these challenges.
Another challenge is to achieve directional propulsion. This requires that an asymmetry arises in the force exerted by the object on the medium. Fortunately, propulsion was successfully realized at an early stage of the research.
On the other hand, the fact that many of the objects did not propel, that there were several types of propulsion and that the objects collided with each other during observation made quantitative measurements impossible. We were able to overcome these problems thanks to the hard work of our collaborators in carrying out the experiments.
According to your research, you mention three different types of propulsion – 'stroke', 'kick' and 'side-stroke.' What determined the style of propulsion?
The style of propulsion was determined based on how an object is deformed. The way an object deforms changes the magnitude, direction and timing of the viscous drag from the water.
What determined the swimming efficiency of the individual crystals?
This is a rather difficult question to answer. There are complex factors involved. In general, crystals with large, elastically moving parts tended to swim more efficiently, but this was not the only factor involved. The flipping frequency and energy acceptance ratio were also involved in the dynamics. An easier way of looking at it is by comparing the crystals to Olympic swimmers. Although Olympic swimmers have a versatile array of swimming styles, there are still similarities in how they swim.
You also created a computational minimum model to understand more about the variables that affected propulsion. What did you learn from this model?
From this model, I learned that directional movement, the second challenge, is, in fact, very difficult to achieve in an ideal environment, as traditionally expected in physics. On the contrary, when we escaped from the requirement of an ideal environment, the directional swimming was calculated to reproduce the experimental results very well.
In other words, the computational model we have described in our paper should not be submitted as a class report on mechanics at university. The fact that we were able to reproduce the experimental results by daring to "deviate from the ideal setting" reminded us that the natural world is made up of a complex interplay of factors.
Your research concluded that a self-sustainable bending crystal can swim directionally in a 2D tank, but it does not swim with high efficiency. What would you say the difference in motion is between 1D, 2D and 3D objects?
Small machines that swim by moving their bodies, albeit in a passive manner, have already been reported. The fact that screwing the S-shaped fins is the best way to make them swim is the thing that Feynman talked about 40 years ago, and there may be no better way to make them swim.
On the other hand, it is difficult to make the screw motion in synthetically created molecular assemblies. Several chemists, including myself, have reported helical deformations of macroscopic molecular assemblies, but they have not yet been able to turn them into autonomous screw motions until now. However, microbial flagella are able to do this, and I believe that chemists will eventually be able to create them.
As for your question about the difference between 1D, 2D and 3D object movement, I think this is a question about the shape of the object, not the tank. There is a trade-off between the shape of an object and the way it can move because of its geometry and the ease with which it can be moved by synthetic chemistry.
The objects with 1D shape have the ability to show screw motion. In the case of a two-dimensional object, the direction of movement is limited to up and down on the surface, a reciprocating motion, and the key to making it swim is to make it move elastically, like flipping a piece of rubber sheet.
In the case of a three-dimensional object, it is not possible to deform it significantly, so it is hoped that swimming will be achieved via the creation of a traveling wave on the surface of the object. I believe that this can be achieved using chemically oscillatory gels.
What are the potential applications of microrobots?
I don't know what the real potential demand is for applications such as autonomous swimming in a tiny space to carry something like drags. An autonomous microrobot is something that has never been created before. But It should be remembered that swimming robots have frequent collisions and are crushed everywhere. We need to fix this issue by making the robot intelligent enough to learn traffic rules.
On the other hand, if we think about the immobilization of the robot, we can see other applications. When we think of the robot in terms of its coordinate axis, the robot makes water move. In other words, our robots have the potential to be used as pumps to move water. From this point of view, we can see potential applications in water purification and artificial dialysis.
The development of molecular robots has attracted considerable attention from physicists and chemists. What does the future of microrobotics look like to you?
I think it is important for humans to dream about what we would like microrobots to do. There have been recent reports of research into micro-robots, including one intended for drug delivery to cancer cells and another on self-replicating and proliferating robots.
In Japan, the ethical issues involved in molecular robotics also seem to be a research subject. As you can see, researchers are thinking a lot about the future of micro-robots, but it seems that the image of micro-robots has not changed much from that of science fiction a few decades ago.
If we can actively think about the future of microrobots in terms of scientific developments, I think that more interesting and useful applications will be born.
What are the next steps for your research?
Our most recent research is to introduce a control mechanism for autonomous swimming. These are molecular robots that are able to swim and stop by reading external signals.
In the longer term, we would like to initiate basic research for social implementation, such as research aimed at improving energy efficiency and creating molecular robots that can function as pumps.
I would also like to create a microrobot that can automatically form when and where it is required and then automatically disappear after it has completed its mission.
About Yoshiyuki Kageyama
Yoshiyuki Kageyama graduated from the college of arts and sciences at the University of Tokyo (Japan) and obtained his Ph.D. from the graduate school of arts and sciences at the same university. His research field is supramolecular organic chemistry, as well as systems chemistry. The synthetic achievements, including the autocatalytic vesicular reproduction system and the self-oscillatory motion of self-assembly by synchronization of an artificial molecular motor, were reported ahead of the rest of the world.
Despite being in the initial stages, the concepts discovered are expected to be key to chemical research in the coming decades. He has been joined five big public projects including "Molecular Technology and New Functions" and "Molecular Engine". He has been working as an assistant professor at the department of chemistry, faculty of science in Hokkaido University since 2009, and leading a very small group as a substantive PI. His contribution to education was awarded as "Excellent Teachers" by the university.
Disclaimer: The views expressed here are those of the interviewee and do not necessarily represent the views of AZoM.com Limited (T/A) AZoNetwork, the owner and operator of this website. This disclaimer forms part of the Terms and Conditions of use of this website.