By Kalwinder KaurNov 28 2012
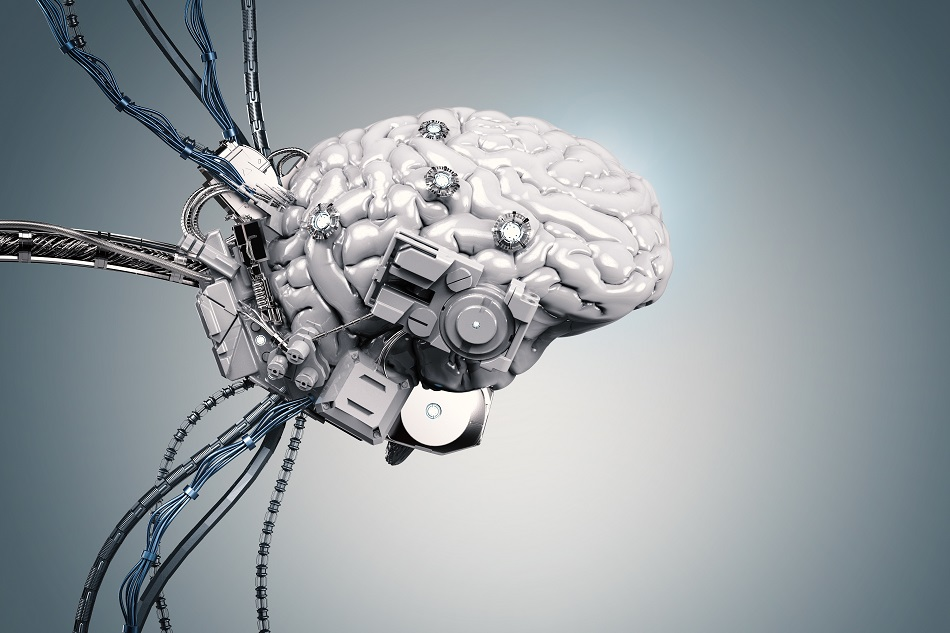
Image Credit: Phonlamai Photo/Shutterstock.com
We are more aware than ever of the inner workings of the brain. Intensive research has contributed to the vast amount of knowledge that we have about the organization and functionality of brain areas. These findings serve as a basis for the development of several novel approaches to resolve central nervous system-related problems such as the restoration of memory loss, and the diagnosis of neural disorders like dystonia and Parkinson’s disease using neural prostheses.
For people suffering from quadriplegia and spinal cord injury, illnesses that limit movements, performed actions do not reflect underlying intentions. In such cases, even simple actions such as holding a glass of water are impossible.
Wearable robots have been introduced to help people with physical impairment but recognizing a user’s intention is still a challenge. Injury causing the loss of limbs during wars has been a major motivation for the attempts to duplicate the hand’s movements with the use of rather crude prostates. The advances in actuators, sensors, and material in the last couple of years allow the replication of the function of the most dexterous extremity: our hands.
Mind Control
Messages are communicated from the brain to the body through neurons in the form of electrical impulses. Neurological disabilities are caused by damage to a part of the central nervous system. BrainGate has introduced a novel technology aiming to improve life for physically-disabled people by enabling them to communicate and interact. The BrainGate neural interface is equipped with a sensor and a brain signal analyzing device. The sensor is implanted in the brain for recording signals. The recorded signals are interpreted and then translated to provide desirable movements.
This technology has allowed people with disabilities to control a computer using thought alone. This happens by detecting the person’s intention through bio-signal sensors. These sensors then detect the electrical signals that the brain transmits to the muscles. Machine learning mechanisms determine the threshold of electrical activity needed to be classified as an intention.
The company has initiated research on making limb movements using the BrainGate neural interface to help people with physical disabilities. Their research is dedicated to designing a thought-controlled wheelchair. The following video demonstrates a breakthrough in the application of the BrainGate neural interface system – a study funded by the National Institute of Health.
Thought control of robotic arms using the BrainGate system
A recent study designed a paradigm for detecting a patient’s intentions for hand robots. By extracting spatial and temporal information using first-perspective, camera users’ intentions were obtained and constructed via the use of a deep learning model. The intentions obtained from the paradigm and the electric signals from the electromyography (EMG) were compared. The intentions detected by the paradigm preceded the patient’s intentions signaled by the EMG. This means that the deep learning model correctly and successfully detected the patient’s intentions to execute a certain movement.
Sensorimotor BCI Technology
The brain-computer interface uses neural input functions to control task-oriented systems. The success of this strategy is highlighted by the implication of BCI in motor movement control. Motor BCI transforms the signal from motor regions of the brain into a command for an external effector to execute, such as robotic arms. This is accomplished by recording the neural activities and then converting them into signals using corresponding transform algorithms for controlling external devices. These algorithms enable the user to switch the device control based on the neural activity.
Movement related features are extracted via input sources that originate in brain regions that have a stable connection with the output functions, including muscle activations and limb movements. Thus, a neural signal can be calibrated from the brain region of a patient who cannot physically execute the movements.
The Future: Cognitive BCI technology
BCI technology could be applied in other areas such as restoring the learning and memory of cognitively impaired patients. However, the physiology underlying the development of BCIs is less likely to be applicable to higher-order cognitive functions such as memory. These functions depend on a dynamic interaction between distributed brain circuits. Thus, choosing a target area to derive an input signal is the main challenge for developing cognitive BCI.
Conclusion
Research has advanced our understanding of the organization and functionality of the nervous system. One of the major goals of modern neuroscience is the restoration of lost function in the cases of memory loss, motor dysfunction and speech disorders. Technology developed to the point of being able to extract an electrical signal from the brain that corresponds to a pattern of activity, decipher these patterns and relate them to a device that can do what the person is no longer able to do. It has improved the life of many physically disabled people. Scientists are on a quest to elucidate the restoration of learning and memory and develop cognitive brain-computer interfaces.
Sources and Further Reading
- Buch, V. et al. (2018). Network Brain-Computer Interface (nBCI): An Alternative Approach for Cognitive Prosthetics. https://www.frontiersin.org/articles/10.3389/fnins.2018.00790/full
- Chapter 2: Basic Anatomy and Physiology of the Human Brain. Published in: Schmidt F.E.W. Development of a Time-Resolved Optical Tomography System for Neonatal Brain Imaging. Med. Phys. 1999: 27, 1343: Pages 1–207.
This article was updated on 6th February, 2020.
Disclaimer: The views expressed here are those of the author expressed in their private capacity and do not necessarily represent the views of AZoM.com Limited T/A AZoNetwork the owner and operator of this website. This disclaimer forms part of the Terms and conditions of use of this website.