Microscopes have been a cornerstone of science for centuries, but they’ve come a long way from the simple lenses of the past. Today, robots are teaming up with microscopes to help researchers do more, see more, and do it all faster. Whether it's spotting early signs of disease or exploring the tiniest structures in our cells, robotic microscopy is unlocking discoveries we couldn't have imagined a decade ago.
This article will break down how this powerful combo is shaking up research, medicine, and more.
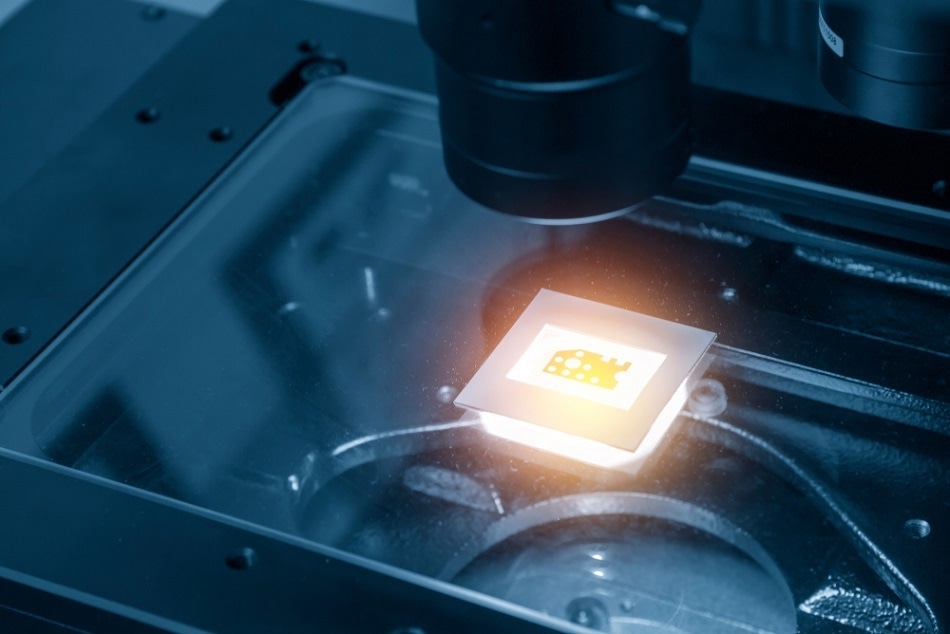
Image Credit: Pixel B/Shutterstock.com
Download your PDF copy now!
Doing More, Faster: Automation and High-Throughput Imaging
Traditional microscopes are amazing, but let’s face it—they can be slow. Manually scanning slides and adjusting focus isn’t just time-consuming; it introduces variability from one user to the next. Robotic microscopes take that out of the equation. They can scan, focus, and capture images automatically, which means researchers can study thousands of samples in the time it used to take to handle just a few.
This kind of scale is a game-changer for long-term studies, like tracking how cells change over weeks or months in diseases such as Huntington’s. Some of these systems produce terabytes of data daily, digitizing everything in real time so researchers can go back and analyze patterns whenever they want.1,2
They’re also a lot more accurate. Robots don’t get tired or distracted, and that means better consistency. In fact, these systems have picked up subtle changes in neurons that humans would likely miss, especially in studies on neurodegenerative diseases. And because every cell gets analyzed on its own, these tools support what scientists call “hypothesis-free” research, where unexpected patterns can emerge from huge datasets.1,2
Tiny Robots, Big Precision
While automation brings scale, miniaturization brings proximity. At the microscale, robotic tools aren’t just extensions of human vision, they become active participants in the system being studied.
A striking example comes from Cornell University, where researchers have created microrobots that are just two microns across. These devices can move through biological samples under magnetic control, acting as dynamic lenses or sensors embedded directly within the tissue.3
Because they operate inside living systems, these robots offer a dual advantage: they can observe and interact at the same time. They’re being used to measure mechanical forces at the nanoscale—something conventional microscopy can’t do in situ. That opens new doors in mechanobiology, helping scientists explore how physical forces shape cellular behavior, development, or disease progression.
In medicine, micro-robotics is beginning to close the gap between diagnosis and intervention. Imagine a robot navigating through the bloodstream, not only identifying cancerous cells but taking a biopsy or delivering a localized dose of medication. Systems like these, inspired by soft robotics and biohybrid engineering, are under active development, and some are already in preclinical trials.4
Multiplexed, Super-Resolved, and Fully Automated
Super-resolution microscopy was already a major leap forward. But it's robotic integration that’s turning it into a scalable, multiplexed tool for studying complex systems.
The maS3TORM platform is a standout. It automates a highly intricate imaging cycle—staining, bleaching, imaging, and restaining—allowing for the sequential visualization of up to 16 protein targets in a single sample. And it does so in 3D, with less than 10 nm localization precision.⁵
Why does that matter? Because biological systems are dense. Proteins don’t act alone; they form networks, architectures, and clusters. Visualizing those interactions in context, without destroying spatial relationships, is essential. Robotic multiplexing gets around the limitations of spectral overlap, enabling scientists to map molecular arrangements in neurons, for example, in a way that preserves biological fidelity.
In practical terms, maS3TORM has revealed previously unknown relationships between motor proteins and synaptic vesicles, suggesting that molecules like myosin might have regulatory functions in neural transmission beyond their traditional roles.5 That’s not just a technical achievement; it’s a conceptual one.
Big Data, Smart Analysis: The Role of AI
All of this—high-throughput imaging, multiplexed super-resolution, mobile micro-robots—generates vast amounts of data. Managing it is a challenge. Extracting meaning from it is even harder.
That’s where machine learning and AI come in. Algorithms trained on robotic microscopy data are increasingly capable of tasks like cell classification, trajectory prediction, and anomaly detection—far faster and often more accurately than human analysts. In neurodegenerative disease studies, neural networks have identified subtle morphological markers in neurons before clinical symptoms emerge.5
AI also plays a critical role in automating lower-level tasks that used to bottleneck workflows: correcting for drift, aligning images, and extracting features. These optimizations aren't flashy, but they're essential for scaling up imaging without compromising quality.
What’s especially promising is the way robotics and AI reinforce each other. The more consistent the imaging conditions, the cleaner the data, and the better the models perform. It’s a feedback loop that’s accelerating discovery across fields.
From Bench to Bedside: Clinical Applications Take Shape
Robotic microscopy is no longer confined to research labs. It’s increasingly being embedded in diagnostic and therapeutic workflows.
- In diagnostics, microrobots equipped with biosensors can detect precancerous lesions in the gastrointestinal tract during endoscopy, with greater sensitivity than standard methods.6
- In drug delivery, magnetically actuated robots can target tumors with chemotherapy agents and verify delivery in real time via imaging. That minimizes systemic toxicity and enables precision dosing.4
- In surgery, robotic systems integrated with real-time microscopy help neurosurgeons differentiate tumor margins from healthy brain tissue, improving resection accuracy.7
These aren’t prototypes—they’re early deployments. And as imaging and robotics continue to converge, the clinical impact is likely to grow.
Microrobots Controlled by Sound and Magnetic Field Deliver Targeted Drug Therapy
Technical Hurdles: Power, Materials, and Data Bottlenecks
That said, the field is far from frictionless. Miniaturization brings its own set of engineering challenges. Powering and controlling microrobots at a distance often requires external magnetic or ultrasonic fields, which can limit mobility and precision. Researchers are experimenting with biohybrid designs—robots propelled by bacteria or algae—but controlling these systems in complex environments remains tricky.3,4
Biocompatibility is another concern. Medical micro-robots need to be non-toxic, biodegradable, and reliably manufacturable. Some systems use polymers that degrade after drug delivery, but making sure they dissolve at the right time and place is still a major challenge.
And then there’s data. With each robot and imaging cycle generating massive datasets, real-time analysis isn’t always feasible. Cloud-based storage and edge computing are helping bridge the gap, but the infrastructure has to keep pace with the tools.
Final Thoughts: Robotics isn’t Just Enhancing Microscopy—It’s Rewriting it
Robotic microscopy isn’t just making old techniques faster or more precise. It’s expanding what microscopy can be. It's enabling scientists to run experiments they couldn’t run before, to ask questions they hadn’t thought to ask, and to answer them with more confidence and clarity.
There’s still plenty of work to do—from building better microrobots to designing smarter algorithms and more robust data systems. But the direction is clear. The microscope is no longer a passive observer. It's an active, autonomous system, one that’s not just showing us the microscopic world, but helping us understand it in entirely new ways.
Want to Explore More Intersections of Robotics and Biology?
Here are a few articles worth reading next:
Download your PDF copy now!
References and Further Reading
- Robotic system monitors specific neurons. Robotics @ MIT. https://robotics.mit.edu/robotic-system-monitors-specific-neurons/
- Tamilselvam Yokhesh, K. (2025). Role of Robotics in the Assessment of Neurodegenerative Disorders. In Current State and Future Perspective in Human-Robot Interaction. IntechOpen. DOI:10.5772/intechopen.1009683. https://www.intechopen.com/chapters/1200863
- Smallest walking robot makes microscale measurements. (2024). Cornell Chronicle. https://news.cornell.edu/stories/2024/12/smallest-walking-robot-makes-microscale-measurements
- Zhang, D. et al. (2023). Advanced medical micro-robotics for early diagnosis and therapeutic interventions. Frontiers in Robotics and AI, 9, 1086043. DOI:10.3389/frobt.2022.1086043. https://www.frontiersin.org/journals/robotics-and-ai/articles/10.3389/frobt.2022.1086043/full
- Klevanski, M. et al. (2020). Automated highly multiplexed super-resolution imaging of protein nano-architecture in cells and tissues. Nature Communications, 11(1), 1-11. DOI:10.1038/s41467-020-15362-1. https://www.nature.com/articles/s41467-020-15362-1
- Alian, A. et al. (2022). Current Engineering Developments for Robotic Systems in Flexible Endoscopy. Techniques and Innovations in Gastrointestinal Endoscopy, 25(1), 67-81. DOI:10.1016/j.tige.2022.11.006. https://www.sciencedirect.com/science/article/pii/S2590030722000885
- Robotics & Imaging Market Research Reports. BIS Research - Market Intelligence on Emerging Technologies. https://bisresearch.com/industry-verticals/Robotics-imaging
Disclaimer: The views expressed here are those of the author expressed in their private capacity and do not necessarily represent the views of AZoM.com Limited T/A AZoNetwork the owner and operator of this website. This disclaimer forms part of the Terms and conditions of use of this website.