Researchers from Cornell University have constructed an artificial cilia system that can control microfluidic flow at an individual level. We speak with Prof. Itai Cohen about his involvement in this research, how these cilia control fluid flow, and further applications these artificial cilia could have in enhancing the healthcare diagnostics and chemical manufacturing industry.
Please could you introduce yourself and tell us about your current position and research activities?
My name is Itai Cohen and I'm a professor of physics at Cornell University; one of the things that my lab is interested in is creating small microscopic robotic systems.
Could you summarize the results of your recent research?
We have managed to create artificial cilia, which in biological systems are little hairs that help to transport fluid. For example, in your brain, cilia line brain cavities and depending on your mood or thought processes, the patterns of the beating cilia will change, altering the trajectories of various chemical and neurotransmitters.
Currently, there is no widely adopted engineering analog for these structures despite the fact that they are major fluid transport structures in the biological world.
Typically, replicating the structure of cilia at the microscale is challenging. How did the team approach overcoming this? Were there any developments particularly instrumental in the successful completion of the artificial cilial system?
All of the developments were heroic, but the first development was creating an actuator that could bend with a radius of curvature of around one micron. We were able to achieve this using a surface electrochemical actuator; here, we developed a platinum thin film that was seven nanometers thin and capped on one side with titanium. When the platinum film is placed under a voltage, it absorbs ions from the solution onto its surface, creating a stress that bends the film.
When you apply the opposite voltage, the ions get driven off and the film returns to its original shape. By oscillating the voltage, you can get this little strip to move back and forth and if you do it fast enough, then you can generate a fluid flow..
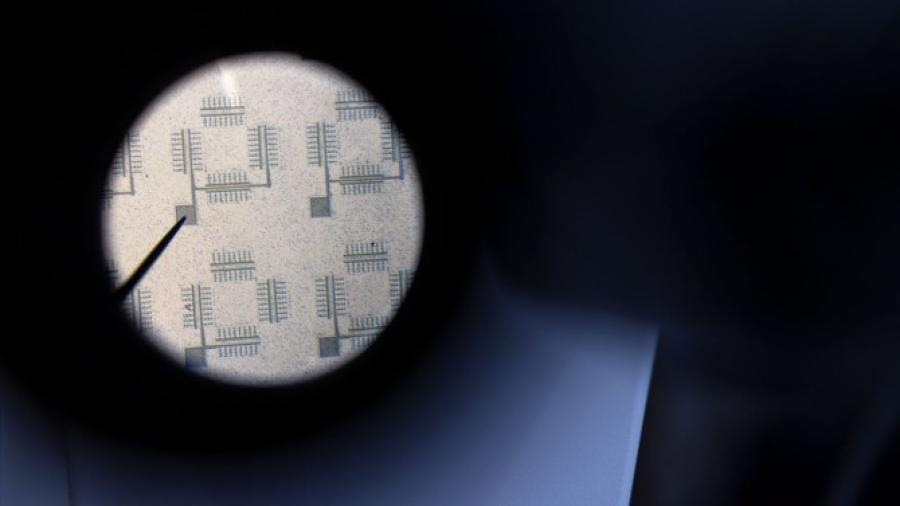
A typical device contains a “carpet” of about a thousand artificial cilia. As the voltage on each cilium oscillates, its surface oxidizes and reduces periodically, which makes the cilium bend back and forth, allowing it to pump fluid at tens of microns per second. © Noël Heaney/Cornell University
A characteristic of this new development is that the cilia can be controlled individually. What are the benefits of this approach?
A promising technology being developed is magnetic cilia that can reorient in an applied magnetic field. However, a limitation of this technology is that it does not give individual controllability. Once cilia can be controlled individually, very interesting flow geometries at the scale of the cilia itself can be achieved.
By selectively activating cilia in different ways, you could generate any elementary flow geometry that you want, and these flow geometries can be put together to obtain any desired flow design/ The idea is that instead of having a uniform cilia carpet doing the same thing, each cilia can generate flows at the microscale which can be pieced together to create any arbitrary flow geometry that you want
Flows could also be switched between one another. For example, you could have a circulating flow that then switches to become a transport pattern.
Each device contains around one thousand artificial cilia; to what extent does the number of cilia influence the degree of flow control?
To establish more flow geometries, you will need more cilia to drive each flow pattern. If you have more cilia, then you can generate more flows on the same device, or you can use the same number of cilia, but just switch between them to change the flow. Both are ways of enhancing the flexibility of the device.
As part of the investigation, a CMOS clock circuit was integrated into the cilia array as a wireless proof of concept. How great is the demand for wireless devices, especially in the field of microfluidic technologies?
Normally, we would have to wire up the cilia one by one, and wire them into a small chip carrier and have a big master computer controlling all the voltages on each of those cilia to drive the different flow patterns. If you could remove this and have a small microchip on board that locally controls what the cilia are doing, and another that controls the next batch, all of these devices could be on one chip around one centimeter on each side.
Fifty years of Moore's Law has made it very easy to create small electronics; the hard part is creating the actuators. Now that we've done that, there is a huge potential for integrating small sensor systems into chips, which could detect chemicals in water or blood. Depending on the sensor's readings, the fluid's flow could be altered.
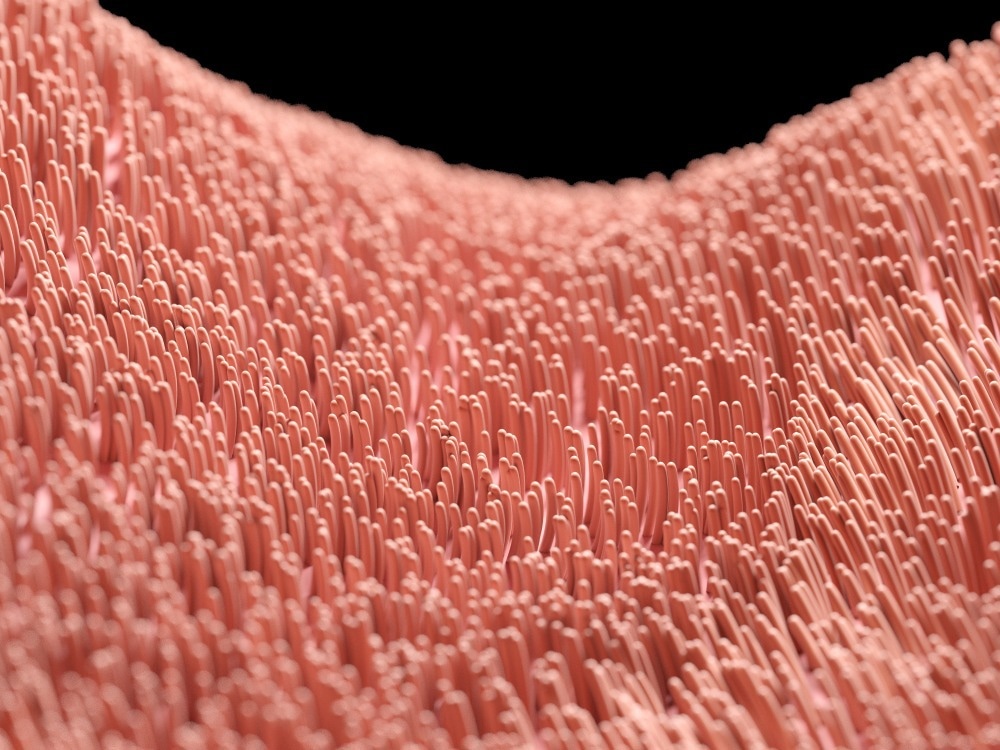
© SciePro/Shutterstock.com
One significant element of the design is that it is low cost, opening the door to more accessible diagnostic testing. How feasible is this, and what would be the steps to accomplish it?
While this is the vision, there are a lot of steps that need to occur before this is a reality. However, it may not be as far as we think. Currently, we are working with commercial foundries to develop these microchips and we are integrating the technology we have been working on.
Moving forward, we are hoping to develop sensors that allow us to detect different chemicals. We want to make the cilia better, by creating cilia with internal structures that allow us to generate flows more efficiently. Synchronizing the chips with one another is another area of research we hope to pursue.
Are there any other applications the cilia could be applied to?
One application we have been discussing is an exciting area of chemistry called flow chemistry. The idea here is that instead of mixing chemicals inside of a big vat and then stirring everything, which is really inefficient, the reactants flow together in small channels.
Imagine if you could have little sensors along those channels that are telling you whether the intermediate products that you're expecting are actually being made, and little responsive cilia altering the flows to enhance these reactions?
To actualize this idea, you would need to move beyond just the cilia and have a cyber physical system where the cilia can communicate with a sort of computer that is modeling both the flow and the chemical reactions. Then, based on these models, the cilia would move to enhance the reaction product.
What has been your favorite aspect of the investigation?
By far, it's interacting with the students, who are putting their heart and soul into the research. Wei Wang led this particular effort and he has been a spectacular student; everything that I can dream up, he's able to manufacture in the lab. He's able to put things together in ways that I didn't even anticipate and brought all of the elements of this research together into this fabulous paper.
It's my privilege to be able to work with fantastic students like this in a collaborative environment like the one that Cornell has to offer. Without Cornell's world-class research, its clean room facility and help from the folks at Cornell nanofabrication facility, we wouldn't have been able to complete our research.
About Prof. Itai Cohen
Professor Itai Cohen studies the physics of matter in motion. At Cornell, his research has focused on building microscopic robots, controlling the shear thickening behavior of microscopic and nanoscopic particles suspended in a fluid, exploring the mechanics of materials ranging from biological tissues to origami inspired metamaterials, discovering the aerodynamic and neuromuscular mechanisms used by insects during flapping flight, and determining how audiences at heavy metal concerts coordinate their movement. Understanding how emergent behaviors arise from the microscopic rules governing these systems remains one of the biggest challenges in Physics.
Professor Cohen received his BS in Physics from the University of California at Los Angeles, and his PhD in Physics from the University of Chicago. Following his graduate studies, he was a Post-doctoral fellow in Physics and the Division of Engineering and Applied Science at Harvard University. In 2005 he joined Cornell and is currently a professor of Physics. Professor Cohen is an NSF Career grant recipient, he is a Fellow of the American Physical Society, and is the recipient of the Kappa Delta Ann Doner Vaughn Award for his work on cartilage mechanics.
He has also served as a Feinberg and Braginsky fellow (2012) and the Rosi and Max Varon Visiting Professor (2021) at the Weizmann Institute and the van der Waals Visiting Professor at the University of Amsterdam (2022). He has published over 110 research articles, given nearly 300 invited seminars, colloquia and conference presentations, and co-authored the book Finding Your Research Voice: Story Telling and Theater Skills for Bringing Your Presentation to Life. His work has been covered by various outlets ranging from the BBC, to NPR, and the NYTimes.
Disclaimer: The views expressed here are those of the interviewee and do not necessarily represent the views of AZoM.com Limited (T/A) AZoNetwork, the owner and operator of this website. This disclaimer forms part of the Terms and Conditions of use of this website.